Abstract
The Mobile Air Conditioning (MAC) contributes with its direct and indirect emissions to the greenhouse effect. The direct effect due the release in the atmosphere of high GWP refrigerant is reducing thanks to the progressive use of low GWP fluids mainly driven by regulations in place in several regions of the world.
The indirect effect, i.e., the CO2 emissions due to the MAC energy demand, is on the other hand not currently submitted to any specific regulations.
The paper gives some data and an assessment of the overall impact of the MAC energy efficiency on CO2 emissions and of the main technologies that are under deployment or that could be deployed to improve it.
Scenario
Road transports are responsible of about the 21% [Khalili, 2019] of global energy demand and account for more than 1.2 billion of circulating vehicles, 950 million of which are passenger cars [OICA, 2022]. The global population is travelling further and more frequently leading to further increase the number up to 2 billion of vehicles on the roads [WEF, 2016].
The energy spent by these vehicles relates not only to motion power (e.g., acceleration, aerodynamics, friction, tires rolling resistance, …), but the energy is also demanded for ensuring comfort and driver safety (de-misting/ de-fogging) and for guaranteeing the operation of all the on-board electronics. In this context, the Mobile Air Conditioning (MAC) represents by far the most energy demanding function.
The average energy demand of a European passenger car travelling at 45 km/h ca. is of about 20 kWh/100km [Pavlovic 2016] leading to an average power demand of approximately 8 kW.
The MAC average energy demand ranges from 1 kW to 3 kW for cooling and from 2 kW to 8 kW for heating as a function of the ambient conditions. This means that the MAC system represents an additional yearly energy demand ranging from 5% up to 10% if also heating is considered as a function of the ambient conditions.
The MAC penetration is close to 100% in advanced economies, while in emerging countries is around 60%, rapidly increasing with the fleet renewal. So, the current global MAC penetration can be estimated, as conservative value, in 80%.
The road transport scenario is rapidly moving towards the full electrification. In Europe there are already very stringent regulations setting the full transport decarbonization in 2050, while other world Regions as North America and China are fast following this path. The electrification of vehicles means that the lack of waste heat form thermal engine needs a replacement for ensuring safety above all (de-misting, de-fogging) and comfort. So, a new generation of thermal systems is under deployment, based on a combination of electric heating and conventional MAC or, preferably, enhancing the MAC functionality with heat pump capability. In any case the thermal system efficiency will be of outmost relevance having it a relevant impact of overall vehicle energy balance and at last on the vehicle effective electric range.
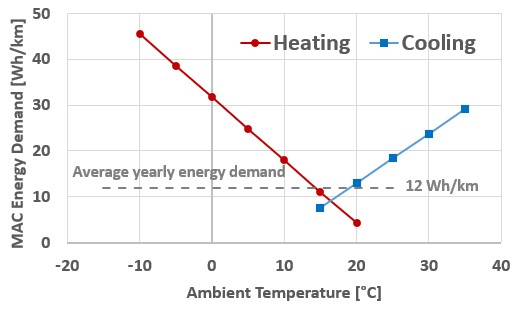
Figure1 – Model of average MAC energy demand function of the ambient temperature in heating conditions [Doyle, 2018] and cooling conditions [Monforte, 2013]. The model is simplified because it does not include the effect of transient energy demand (passenger compartment warm up and cool down) and of solar load and only partly include the effect of relative humidity [Monforte, 2013].
MAC energy demand
Among the several studies on MAC energy demand available in the recent literature, two have been selected to support the creation of a simplified model for the MAC energy demand in heating and cooling conditions.
The following analysis will focus on Light Duty Vehicles (LDV, passenger cars and vans) that represent by far the largest number of circulating vehicles.
Considering that:
- the average LDV energy demand (WLTP testing cycle) is of about 200 Wh/km [Pavlovic 2016],
- the yearly energy demand of a modern MAC is about 6% of the vehicle energy demand (i.e., 12 Wh/km) for cooling [Bloomberg, 2019],
- as simplified assumption, an additional 6% should be considered for ensuring heating [Bellocchi, 2018] in case of electric car due to the lack of waste thermal heat from the engine,
- the yearly average travelled distance is of 20’000 km [EUCOM, 2018], [US DoE, 2020],
a single MAC will demand approximately 480 kWh (50% for cooling and 50% for heating) per year for ensuring the thermal comfort and the other related functions comfort under the above listed conditions.
Then, using the Willans factors average [EUCOM, 2018], 0.25 l/kWh to convert the energy in liter of fuel for thermal engine-powered vehicles, a MAC needs 60 l of fossil fuel (i.e. petrol, diesel, natural gas, LPG, …), whose combustion produces (2550 gCO2/l) 150 kgCO2, per year.
In case of a pure electric vehicle and using the world electric energy CO2 equivalent [IEA, 2019] of 475 gCO2/kWh the MAC energy demand for cooling is of about 114 kgCO2/year. This value almost doubles i.e., 228 kgCO2/year, adding the need of heat power to compensate the lack of waste heat from the thermal engine and under the above listed assumptions.
So, the indirect CO2 eq emissions are larger than the effect of direct emission (refrigerant leak during manufacturing, use and end of life) in case of use of R-134a (GWP 1430), see Table 1. The direct emissions of a R-134a-based system have been estimated to be 500 kgCO2e for the whole lifetime [Bloomberg, 2019], assumed to be 15 years. Consequently, the direct average direct emissions per year are of about 33 kgCO2e/year.
Considering that there are approximately 1’000’000 of Light Duty Vehicles circulating and assuming a worldwide penetration, the overall CO2eq emission due to the MAC use are of about 15 million of TonCO2eq in case all vehicles are equipped with a thermal combustion engine or 23 million of TonCO2eq in case all vehicles are full electric.
These figures highlight the relevance of the MAC efficiency and even more point out that the MAC efficiency is even more important for fully electric vehicles where ethe lack of waste heat from thermal engine.

Technologies for increasing the MAC efficiency
The MAC energy efficiency can be improved combining the effect of several possible actions:
- Electrical (fan, blower, …) and mechanical (compressor, heat exchangers) components efficiency enhancement
- System architecture: direct expansion vs indirect expansion, water cooled condenser, internal heat exchanger, heat pump vs electric heater
- Improving the vehicle characteristics to reduce the thermal load: improved insulation, window coatings and reflective paints.
These combined energy efficiency improvements could reduce the energy consumed by MAC up to 65% [IEA, 2019b].
The following chapter will focus on two specific issues: the effect of the use of an heat pump architecture for ensuring the winter heating in battery electric vehicles and the effect of refrigerants on MAC efficiency.
Heat Pump
The electric vehicle (BEV – Battery Electric Vehicles) or the hybrid vehicle with relevant electric range capability (PHEV – Plug in Hybrid Vehicle) requires specific measures to ensure comfort and safety function (de-misting and de-fogging) in mild and cold weather conditions due to the lack of waste high temperature heat that is usually available on the conventional thermal engine-powered vehicles (ICEV – Internal Combustion Engine vehicles).
The simpler solution is an electric (PTC – Positive Thermal Coefficient) heater. A purely dissipative device transforming the electric energy stored in the battery in heat that is transferred directly or indirectly (heat-to-liquid and then with a conventional liquid to hear heat exchanger) the passenger compartment. Typically, a PTC heater as an average power of about 6 kW, ranging from 3 kW up to 10 kW, as a function of the vehicle characteristics.
The heat pump (HP) is a configuration of a MAC system, that usually transfers heat form the passenger compartment to the ambient, enabling to transfer of heat from the ambient to the passenger compartment.
This function can be performed with a conventional direct expansion system, reversing the thermodynamic cycle [Kowsky, 2012] or by-means of a dual loop cooling system [Optemus, 2019, Ferraris, 2020] . In this case the thermodynamic cycle remains unchanged, and the reverse function is actuated by reversing the coolant flow.
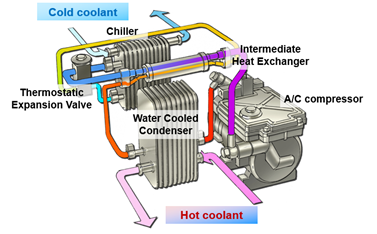
The heat pump allows to reduce the energy demand for ensuring heating in a very relevant way. In heating mode, a typical heat pump system used in place of PTC heater enables an energy saving ranging from 17% up to 52%, depending on the geographical context and on the employment of an ERV-equipped system [Bellocchi, 2018].
Refrigerant
Today, nearly all LDVs in Europe, Japan, North America and in some other countries use ozone-safe hydrofluoroolefin (HFO)-1234yf with GWP = 4 as refrigerant. In 2020, four European brands introduced R-744 heat pumps in some of their high-volume electric vehicle models, and SAE International’s Interior Climate Control Committee updated its standards to allow the R-152a use in secondary loop MACs (SL-MACs).
The refrigerant itself slightly affects the MAC efficiency, while the system requirement associated to a given refrigerant could have a relevant impact on final energy demand. To achieve the same performance levels in case of the use of HFO-1234yf, manufacturers either apply an IHx (Internal Heat Exchanger) or a larger degree of sub-cooling while, for R-744, an appropriate system design (always including the IHx) and specific controls are required [Malvicino, 2009; Zilio, 2009; Strupp, 2011; Koehler, 2018; Westerloh, 2019].
Worldwide regulation scenario
The requirements to reduce the MAC indirect GHG emission are now global via the Kigali Amendment, while the policies addressing the MAC efficiency, generally giving credits to more efficient MAC, are currently implemented in some regions.
In the next future is foreseeable that the number of countries enforcing measures to promote the MAC efficiency will increase, because the CO2 emission reduction has become a global priority, as well as the need to reduce the energy demand. In this framework, a crucial element is to set-up a reliable, reproducible, and possibly low effort demanding standard testing procedure, either as part of the overall vehicle energy test or for the MAC system alone.
U.S. standards rely on credits to incentivize MAC efficiency improvements and load. The EPA recently introduced the AC171 cycle, which is required to verify credit applicability for MAC systems and thermal technologies. Canada LDV GHG standards are aligned with US and Mexico is going to implement a credit mechanism specific for MAC systems.
The European Commission’s standard (EU 631/2019) will allow to account as “eco-innovations” the improvements to MAC systems from 2025.
Conclusions
A simple and conservative approach has been used to assess the impact of the MAC (cooling and heating functions) on vehicle energy balance and overall CO2 emissions. The MAC system requires at least 6% of vehicle energy demand (i.e.12 Wh/km) for ICEV; this approximately doubles in case of BEVs for ensuring the heating function due to the lack of waste heat form thermal engine generating from 15 TonCO2eq to 25 TonCO2 eq per vehicle per year, considering the average world energy mix for electricity.
The vehicle global fleet will grow from 1.2 to 2 billion vehicles in 2040 and the MAC system penetration will very likely reach 100%, being already at this level in the most developed regions of the globe and rapidly increasing from the around 60% current level in the rest of the world.
So the MAC system is responsible of 18-27 millions of Ton CO2eq, that could become around 45 Millions of Ton CO2 eq in 2040 without the deployment of any improvement.
The MAC energy efficiency, when operating in cooling mode, can be improved combining the effect of several possible actions up to 65%, while the use of efficient heat pump can reduce the need of energy in heating mode up to 52%.
Even if only a part of the possible improvement will be deployed, there will be the possibility to at least contain the energy demand increase offsetting the effect of fleet increase.
The industry, partly driven by the need of reducing the vehicle CO2 emission and to save the nominal range of an electric vehicle, and partly to follow the indication from the different regulatory framework, is progressively improving the efficiency of MAC system. The followed path is the deployment of the here listed technologies and the progressive use of HP system for BEVs.

Carloandrea Malvicino
References
Bellocchi S., Guizzi G. L., Manno M., Salvatori M. Zaccagnini A., 2018. Reversible heat pump HVAC system with regenerative heat exchanger for electric vehicles: analysis of its impact on driving range. Applied Thermal Engineering Volume 129, 25 January 2018, Pages 290-305. Available at https://www.sciencedirect.com/science/article/abs/pii/S1359431117338875
Blumberg K., Isenstadt A., Taddonio K. N., Andersen S. O., Sherman N. J. , 2019 Mobile air conditioning: the life-cycle costs and greenhouse-gas benefits of switching to alternative refrigerants and improving system efficiencies. ICCT 2019. Available at https://theicct.org/publication/mobile-air-conditioning-the-life-cycle-costs-and-greenhouse-gas-benefits-of-switching-to-alternative-refrigerants-and-improving-system-efficiencies/
Doyle A., Muneer T., 2018. Energy consumption and modelling of the climate control system in the electric vehicle. Energy Exploration & Exploitation 0(0) 1–25. Available at https://journals.sagepub.com/doi/full/10.1177/0144598718806458
EIA, 2021, World net electricity generation by source. https://www.eia.gov/outlooks/ieo/pdf/IEO2021_ChartLibrary_Electricity.pdf
EUCOM, 2018. Technical Guidelines for the preparation of applications for the approval of innovative technologies pursuant to Regulation (EC) No 443/2009 and Regulation (EU) No 510/2011. Available at https://ec.europa.eu/clima/policies/transport/vehicles
Ferraris, W., Bettoja, F., Casella, M., Rostagno, M. et al., 2020. Heat Pump for BEVs: Architectures and Performance Analysis. SAE Technical Paper 2020-37-0030, 2020, doi:10.4271/2020-37-0030.
IEA, 2019a Report extract Emissions. Available at https://www.iea.org/reports/global-energy-co2-status-report-2019/emissions
IEA, 2019b, Cooling on the Move The future of air conditioning in vehicles Available at https://www.iea.org/reports/cooling-on-the-move
Koehler, J., Westerloh, M., Twenhoevel, S., Tegethoff, W., Schumacher, W. 2018. Worldwide Electrical Energy Consumption of various HVAC systems in BEVs and their Thermal Management and Assessment – Control Architecture and Results. SAE,TMSS Thermal Management Systems Symposium, San Diego, CA, USA, October 9-11, 2018
Kowsky C. Wolfe, E., Leitzel L., Oddi F., 2012. Unitary HPAC system. SAE International Journal of Passenger Cars – Mechanical Systems 5(2):1016-1025
Malvicino C., Seccardini R. 2012. Thermal Systems Integration for Fuel Economy – TIFFE -Transport Research Arena – Europe 2012, http://www.tiffe.eu/
Malvicino, C, 2009, The 4 Pandas experiment: LCCP comparison, 3rd Eu WS on MACS&Aux. Proc., Turin 2009
Monforte, R. and Mandrile, M., 2013, Effects on Real Life Fuel Efficiency of Raising the MAC Engagement Temperature, SAE Int. J. Passeng. Cars – Mech. Syst. 6(2):1021-1029, 2013, https://doi.org/10.4271/2013-01-1506.
OICA, 2022. Motorization Rate 2015 – Worldwide https://www.oica.net/category/vehicles-in-use/
Optemus, 2019, EU funded project. Available at www.optemus.eu
Pavlovic J; Marotta A; Ciuffo B. 2016, CO2 emissions and energy demands of vehicles tested under the NEDC and the new WLTP type approval test procedures. APPLIED ENERGY 177; 2016. p. 661-670. JRC101808. Available at https://www.sciencedirect.com/science/article/pii/S0306261916307152
Siavash Khalili, Eetu Rantanen, Dmitrii Bogdanov and Christian Breyer , 2019, Final global transport energy demand in absolute (left) and relative (right) values for the years 2015 to 2050. Energies 2019, 12, 3870. Available at https://www.researchgate.net/figure/Final-global-transport-energy-demand-in-absolute-left-and-relative-right-values-for_fig3_336516712
Strupp, N. C. 2011. Betriebsverhalten von Verflüssigern in automobilen Kältekreisläufen. PhD Thesis. University of Braunschweig, 2011.
US Dept of Energy, 2020. Average Annual Vehicle Miles Traveled by Major Vehicle category, https://afdc.energy.gov/data/10309
WEF 2016, The number of cars worldwide is set to double by 2040. https://www.weforum.org/agenda/2016/04/the-number-of-cars-worldwide-is-set-to-double-by-2040
Westerloh, M. 2019. Analyse des weltweiten Energiebedarfs zum Heizen und Kühlen von Elektrofahrzeugen. PhD Thesis, University of Braunschweig, 2019
Zilio, C. 2009. A small vehicle MAC system exploited w/ R-1234yf: bench results. 3rd MAC Workshop, Turin, Italy, 2009.